WTF are RNA Vaccines?
- Elena Lopez
- Aug 13, 2020
- 11 min read
Why is RNA unstable?
1. RNA is prone to base-catalyzed hydrolysis due to a hydroxyl group in the 2’ carbon position.
RNA contains a ribose sugar (has 5 carbons labeled below) which has a hydroxyl (-OH) group at the 2' carbon position. This feature makes RNA chemically unstable compared to DNA, which does not have a 2' hydroxyl group, hence the term deoxyribose (the ‘D’ in DNA). The electron lone pair on the nucleophilic (electron donating) oxygen in the hydroxyl group attacks the electrophilic (electron accepting) phosphorus (has an empty bonding orbital) to carry out an intramolecular reaction to hydrolyze (break apart) the RNA sequence. A base (usually a negatively charged hydroxide in aqueous conditions due to the auto-ionization of water) can deprotonate the hydrogen from the 2’ hydroxyl group (-OH) to leave an alkoxide (oxygen with a negative charge), an even more potent nucleophile to attack, hence the term base-catalyzed hydrolysis. In summary, a nucleophile (base) makes a weak nucleophile (hydroxyl group) into a stronger nucleophile (alkoxide) to better attack the electrophile (phosphorus). Resonance stabilization and electronegative (electron greedy) oxygens stabilize the negative charge in the phosphate group. More generally, RNA is weaker than DNA since it lacks base stacking, transient van der waals interactions and hydrogen bonded complementary bases characteristic of DNA.
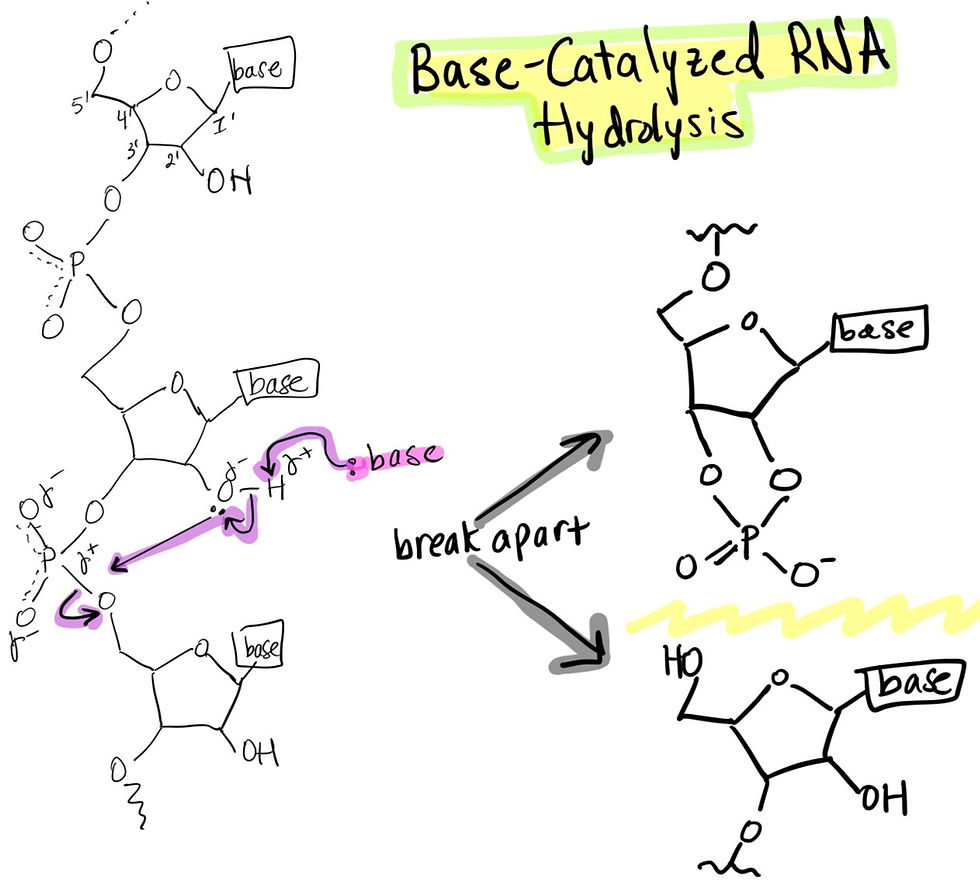
Evolutionarily, it makes sense for DNA to be more stable than RNA since we want to ensure the genome that dictates all future generations of cells remains intact and as stable as possible! RNA is like an interpreter of DNA that allows for messages (genes) to be expressed in an accessible and regulated way (epigenetics).
2. Enzymes may degrade RNA in the cytoplasm.
Human RNA matures when post-transcriptional modifications are added. A 5’ cap and a 3’ poly-A tail serve as the ‘armor’ to protect mRNA from the treacherous environment that is the cytoplasm.
The cytoplasm has an abundance of RNAses and exonucleases, enzymes that chew up and degrade RNA. There are several pathways that play a role in this degradation. These pathways exist as a control of gene expression. A gene may have been transcribed, however, negative feedback mechanisms, cell-cell communication or environmental signals may trigger a limit to protein production. By degrading RNA, an extra level of control before translation of proteins is allowed. The deadenylation dependent mRNA decay pathway, for instance, gradually shortens the poly-A tail of the mRNA and involves the recruitment of de-capping enzyme complexes.
3. Innate immune receptors, such as TLRs and RIG-I receptors, may detect and mount an attack on nucleic acids in the cytoplasm of the cell.
How does your body know to differentiate between itself and other, foreign components? Well, that is the role of the immune system! The innate immune system is the most primitive, essential and conserved form. Pattern recognition receptors (PRRs) in the innate immune system act as sensors of pathogen-associated molecular patterns (PAMPs), usually expressed by a broad range of pathogens. The innate immune system, unlike the adaptive, is nonspecific. These patterns are generalized rules of thumb that hint that something foreign should “not be there”. Activation of these receptors triggers a downstream signalling cascade to activate transcription factors, such as NF-kB and IRF3, that express cytokines and interferons, respectively. Cytokines recruit more cells to the site of infection, increasing inflammation and antigen presentation to adaptive immune cells. Interferons, secreted signalling proteins, play a central role in the antiviral response through different mechanisms, such as reducing protein translation.
Toll-like receptors (TLRs) are a thoroughly studied family of PRRs that notice common pathogenic antigens from viruses to fungi to bacteria. These receptors can be found on cytoplasm endosomal membranes or on the cell membrane to detect intracellular and extracellular antigens, respectively. The antigen binding domain on the N terminus of the receptor contains leucine-rich repeats (LRRs) which form a horseshoe structure, while the C terminus of the receptor triggers the signalling cascade.

Merck therapeutics is currently undergoing clinical trials on a TLR7 and 8 inhibitor as a potential treatment for the cytokine storm in severe, fatal cases of COVID-19. These TLRs can detect single stranded RNA from SARS-CoV2. By inhibiting the detection of the coronavirus ssRNA, one can dampen the inflammation that leads to cell death in COVID-19.
Retinoic acid-inducible gene I (RIG-I) receptors are cytosolic sensors of viral RNA species, important in innate, antiviral responses. Opposite to TLRs, the N terminus is responsible for signalling and caspase recruitment, while the C terminus is the antigen binding domain!
Host mRNA is differentiated by these immune receptors from pathogenic ssRNA based on whether the RNA sequence has the 5’ cap and poly-A tail modifications, a very clever distinction.
All in all, although innate receptors for nucleic acids in the cytoplasm such as TLRs and RIG are well studied, the exact mechanism of how RNA vaccines trigger an immune reaction remains unknown.
What is the basic structure of an RNA Vaccine?
The only knowledge needed to generate an RNA vaccine is the genetic sequence of the pathogen of interest. RNA vaccines are durable, amplifiable and simple to engineer and manufacture. As previously discussed, the RNA drug itself can be recognized by immune receptors so it can simultaneously act as an adjuvant (danger signal) and a foreign coded antigen! Since the recipe for an immune response is to deem an antigen both foreign and dangerous, this accomplishes both checkpoints!
The process begins with in vitro transcription (aka, transcription ‘in a dish’). For this you need:
Linearized plasmid DNA: genetic sequence encoding the vaccine antigen’s sequence of interest
Recombinant RNA polymerase: to transcribe the DNA and make the mRNA
Nucleoside triphosphates: to constitute the RNA that will then be encapsulated for delivery to a host
After the RNA sequence is generated, a 5’ cap and 3’ poly-A tail are added to mimic a mature, mammalian mRNA sequence. This would quell TLR detection, since TLRs, as previously mentioned, tend to distinguish RNA without these modifications. Notwithstanding, the 5’ cap is actually recognized and bound by the small subunit of the ribosome to jumpstart translation of proteins. One can use FPLC and HPLC purification methods to ensure there are no immature mRNA (uncapped, no poly-A tail) remaining after the modifications are added. Otherwise, immature mRNAs could contaminate and inhibit translation by activating immune receptors such as TLRs. These developments allowed for the genesis of the conventional RNA vaccine approach.
Alternatively, alphavirus genomes can be engineered to generate an alternative: self-amplifying RNA vaccines. The genome sequences coding for alphavirus structural proteins are deleted and replaced by the gene of the antigen of interest (like a cut-and-paste approach!). The replicase sequence coded by the alphaviruses directs its own synthesis, mimicking production of antigens in vivo by viral pathogens, triggering humoral and cellular immune responses. This makes for a more potent vaccine: same protection, lower dose, longer lasting effect.
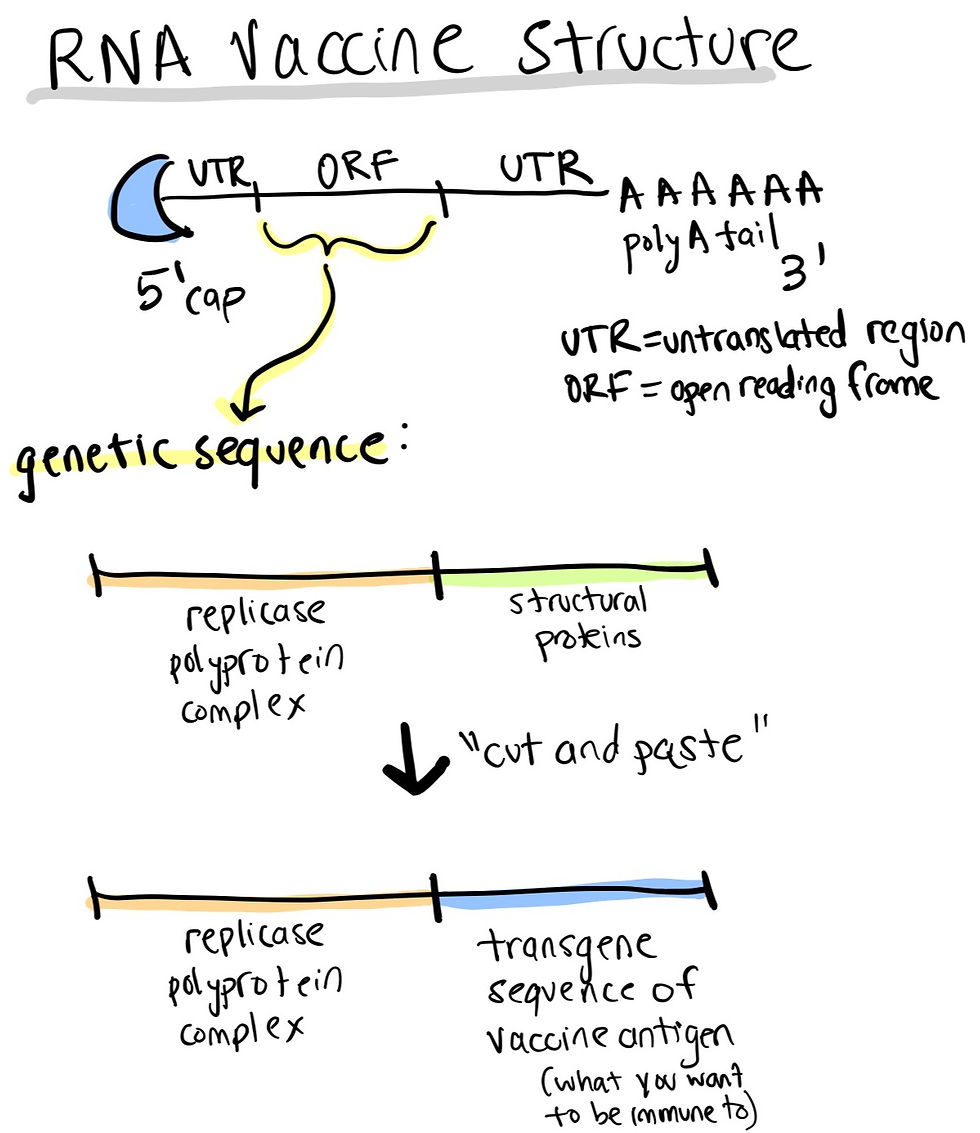
How can RNA vaccines be modified and optimized?
Delivery and formulation of RNA vaccines are important for:
vaccine kinetics
potency of immune response
magnitude of antigen expressed
The goal of delivery vehicles is to:
overcome the cell membrane barrier
allow the RNA to escape the endosome
protect the RNA from enzyme digestion inside the cell
Some ways to optimize RNA vaccines include:
Encapsulating RNA in cell penetrating, cationic peptides and lipids: protects mRNA from degradation and acts as a powerful danger signal to induce an adaptive immune response (T cells and antibodies). With lipid coats, mRNA vaccines are also more thermostable (resilient to temperature changes).
Modifying nucleoside bases on RNA: prevents the folding of the RNA onto itself for the immune system to attack. This allows the RNA to remain intact long enough for enough antigen to be produced to induce long term memory. Examples of modifications include pseudouridine or 5-methylcytidine.
Add post-transcriptional modifications, such as a 5’ cap and poly-A tail to the RNA: prevents premature degrading of the RNA by mimicking human, host mRNA
Purification of RNA product using High Performance Liquid Chromatography (HPLC): eliminates contamination of RNA product that did not receive the intended modifications
What is the advantage of RNA over other types of vaccines?
Traditional Vaccines involve a complex, long, costly process of production, with relatively limited flexibility to adjust immune responses using adjuvants. More so, not much is known regarding the mechanisms of efficacy; most interpretations are based on outcome after vaccination.
In contrast, nucleic acid vaccines hold several advantages:
Production is easier: they are cell-free, simple, standardized, cheap, cold-chain free and rapid, compared to whole microbe, live-attenuated and subunit vaccines. The sequence of the virus, coding all of its antigens, is the only thing needed to generate the vaccine. Hence, how Moderna Therapeutics was able to make an mRNA vaccine ready for clinical trials just 10 weeks after the publication of the genetic sequence of SARS-CoV2. Sequencing and purification of the standardized product allows for convenient, fewer, less precisely controlled steps in manufacturing.
Extra Adjuvant of ssRNA: can mimic natural viral infections to induce broader, potent immune response, although mechanisms remain unknown. Unlike traditional vaccines, one can also titrate and decide which specific antigens are delivered through the coded RNA sequence. This flexibility can allow one to fine-tune the balance between humoral and cellular immunity induced from the vaccine.
Can target specific, conserved antigen domains to target a broader class of pathogen: the only thing better than targeting an antigen specific to a particular bacteria, for example, is targeting an antigen that is common and specific to a whole family of bacterial species! If that family had 10 species, for instance, that would be like killing 10 birds with one stone!
Public health and commercial advantages: Development of thermostable vaccines has been gaining traction and attention, since worldwide, efficient distribution of biodegradable, delicate vaccines are not practical in the context of global health. Poor rural areas or tropical countries may not have the resources to keep large stocks of vaccines frozen for extended periods of time. Modifications, as aforementioned, are a unique advantage that allow RNA vaccines to circumvent these issues by being engineered to be adaptable.
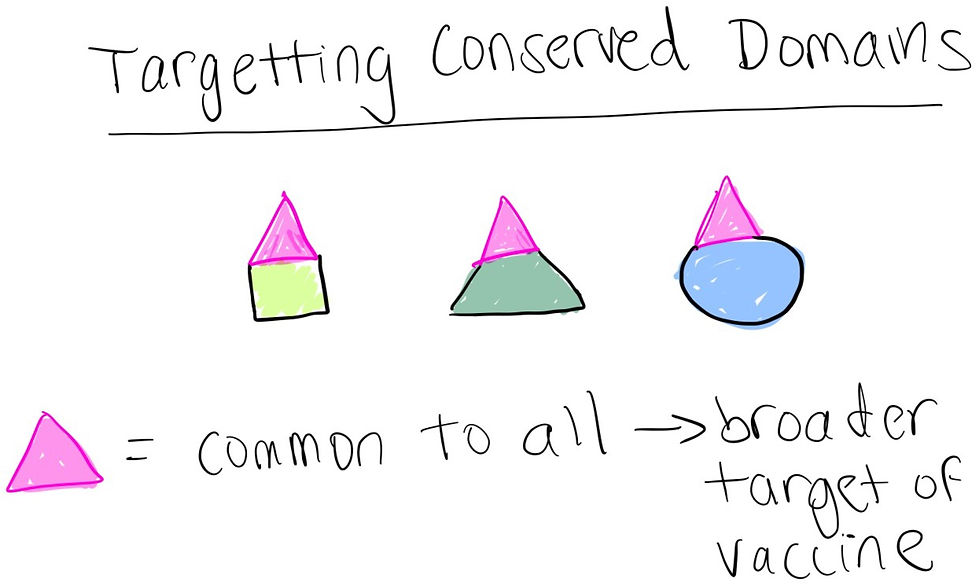
Nucleic acid vaccines, DNA or RNA, deliver a nucleotide sequence that codes for proteins that pathogens use to cause disease. The goal is for the injected sequence to be translated by the host cell into proteins the body will recognize as foreign and dangerous, mimicking a native infection to elicit an immune reaction (albeit without the ability to cause disease or spread contagiously).
DNA vaccines present several challenges, compared to RNA vaccines:
DNA needs to penetrate not only the cell membrane, but also the nuclear envelope to enter the nucleus. In order to cross more membranes, the dosage is often much higher and the delivery is more complicated. RNA only needs to cross one membrane, the cell membrane, to enter the cytoplasm, so lower, less toxic concentrations can elicit a stronger response.
DNA requires an extra step of transcription in the nucleus before producing the vaccine antigen in the cytoplasm. RNA can skip a step of transcription so that it can be directly translated into proteins!
DNA vaccines have the risk of permanently changing the host’s DNA. Newsflash, our genome is DNA so we do not want something similar integrating into it... I, for one, wouldn’t want to have daughter cells producing a foreign pathogen protein. Luckily, research shows this is not very likely.
Nevertheless, DNA does have an advantage over RNA vaccines. It can be harnessed to make not one, but MANY copies of mRNA. Thus, DNA allows for a more potent dose to better activate the immune system. Since memory is the basis of why vaccine logic exists in the first place and a stronger immune response is associated with stronger memory, scientists were drawn, like vultures to a possum carcass, to find a way to engineer RNA vaccines to have these benefits as well. This promise led to rising interest in self-amplifying RNA vaccines.
After all, more potency of vaccines could have great implications, including:
less number of doses needed for desired effect
more effect for less concentration
less risk of toxicity due to volume delivered
How has RNA vaccine development evolved over time?
Past: DNA Plasmids
In the early 1990s, injecting nucleic acids (RNA or DNA) into the muscles of mice led to in vivo expression of the proteins the nucleic acids coded for. These foreign proteins, a component of the evil pathogen, were enough to trigger an immune response by the host who received the injection. This opened doors to a brand new realm in vaccinology. Gone were the days vaccines could only consist of ‘dumb’ versions of the original virus. A simple genetic sequence of a specific domain of the virus alone was sufficient to induce the immunity that would grant a rapid response upon secondary exposure to the real, whole virus. By using nucleic acids, our own cells can be the factories to translate the protein, eliminating a lot of complexity otherwise entailed in manufacturing.
Initially, delivery of the genetic sequence was in the form of a DNA plasmid (small, circular extrachromosomal DNA), but later advances in delivery and the addition of sequences to stimulate the immune system to ramp up even more (genetic adjuvants) made the vaccines even more efficient. This was accomplished by increasing mRNA stability and protein production.
Present: Self Amplifying RNAs
As previously mentioned, self amplifying RNA vaccines sit at the crossroads between the convenience of RNA and the amplifiable potential of DNA as vectors. These self-amplifying RNA vaccines are derived from an alphavirus genome. The genetic sequence of these include 2 components: the sequence coding for the replication, polyprotein complex and the transgenic sequence encoding the vaccine antigen in lieu of all the structural proteins you would normally find in a virus genome. This is a classic “if you cannot beat the virus, become the virus” approach.
Self-amplifying RNA vaccines can be delivered in 1 of 3 ways in order to elicit a stronger immune response than mRNA alone:
DNA Plasmid: The replicase and transgenic sequences are coded into a circular DNA vector which is easy to manufacture. The plasmid enters the nucleus of the host cell, where the DNA is transcribed into positive sense RNA. The replicase sequence codes for a replicase enzyme that converts the positive sense RNA into negative sense RNA sequence. Negative sense RNA can then be translated by host ribosomes to translate the protein that will elicit an immune reaction.
Virus-like RNA particles: The RNA linear sequence is encapsulated in a safe viral coat which enters the cell through endocytosis. Released from the encapsulated endosome, the RNA sequence escapes into the cytoplasm to produce the vaccine antigen trigger similarly to the plasmid DNA approach.
This kind of approach reminds me of a pseudovirion, since the nucleic acid and coat of the delivered ‘virus’ are mismatched. In other words, the viral coat is from another virus entirely; the proteins coating it are not coded by the genetic sequence the viral particle carries within it.
In vitro transcribed RNA: This option allows for the RNA linear sequence to be delivered in a synthetic formulation (lipid nanoparticles, for example). In vitro transcribed means the positive sense saRNA is generated on a dish before it is delivered/injected into a host. The vaccine antigen is produced similarly in the cytoplasm.
This is a very attractive approach for several reasons:
Self-amplification ability of the replicase allows for more copies of the transgenic mRNA sequence
Potential to include immunogenetic factors to induce an even stronger immune response and therefore memory
Synthetic delivery allows for large scale production of formulations
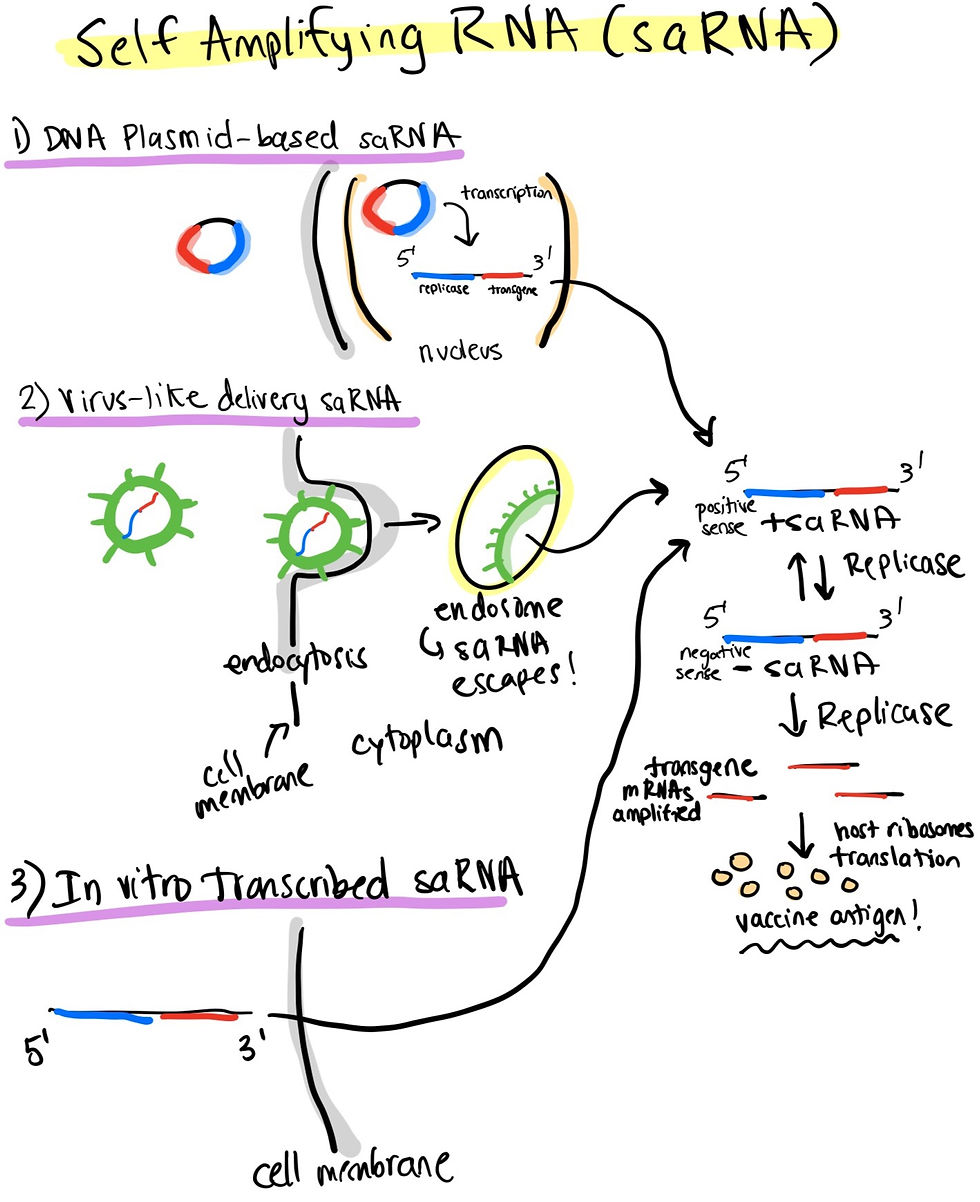
Future: Trans Amplifying RNAs
Alternatively, the most cutting edge recent developments in RNA vaccines are termed trans-amplifying RNAs, a novel bipartite system.Trans amplifying is just as efficient as self-amplifying RNA despite the separate delivery. It is called “in trans” since 2 genetic RNA sequences (the replicase and vaccine antigen sequences) are delivered simultaneously, yet separately (not on the same linear sequence).
The antigen RNA sequence is generated from a self-amplifying RNA from which the replicase RNA sequence is deleted to form a transreplicon. Replicase activity is provided either by a standard saRNA or an optimized non-replicating mRNA (nrRNA), separately. The latter is the preferred delivery since nrRNA can be optimized to amplify the transreplicon sequence significantly more than saRNA.
Wait, isn’t this analogous to “complementation” in virology?
Complementation is when 2 strains of a virus infect a host cell, but 1 of these strains is nonfunctional/mutated. The normal virus helps the mutant, ineffective virus by making protein for both viruses. In the trans amplifying RNA approach:
The mutated, nonfunctional virus = the transreplicon, the saRNA sequence that codes for the vaccine antigen
The normal virus = the replicase delivered as a separate nrRNA sequence, which helps convert positive into negative sense RNA to be directly translated by host ribosomes into the immunogenic antigen of interest
Advantage of Trans amplifying over Self amplifying RNA:
More safe: prevents the risk of exocytosis and both sequences entering a new host cell together
Easier optimization: allows fine tuning of the mRNA to replicase ratio, although how much is titrated to each cell is hard to control, but studies show the same magnitude of effects as self-amplifying RNA vaccines anyway (in which case, the ends really do justify the means!).
Increased manufacturability: it is easier to produce shorter RNA transcripts rather than one longer RNA transcript (as in the self-amplifying RNA approach).

Thank you for reading and please subscribe if you enjoyed :)
Follow @immunethought on Instagram for post updates!
Comments